Fiber Cavity coupled Silicon-Vacancy Center for a Quantum Repeater Node
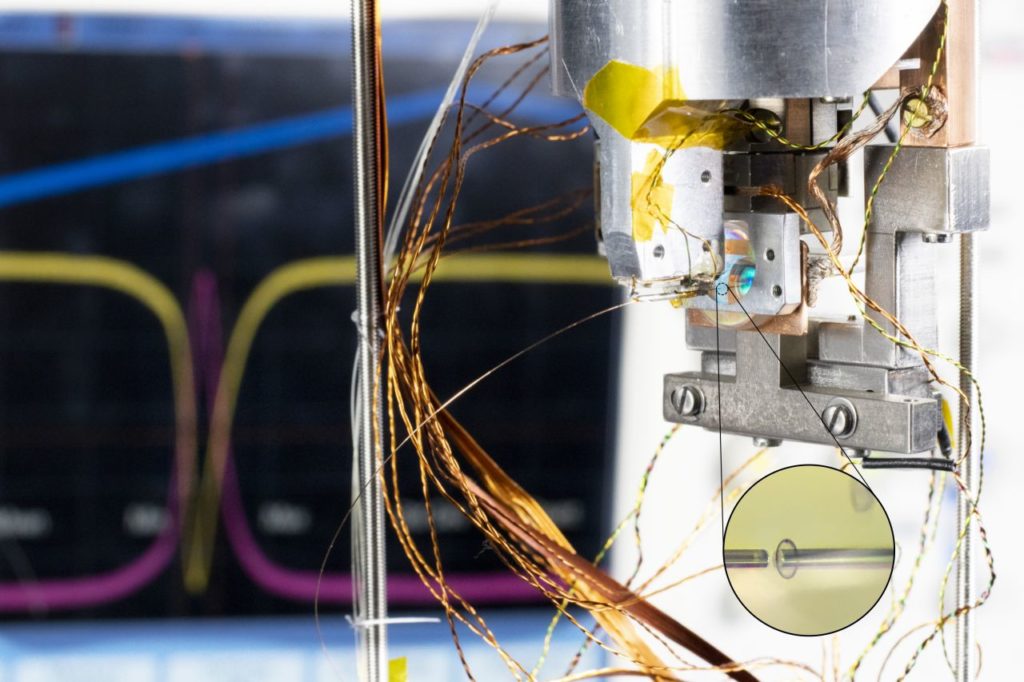
A collaboration of more than 25 institutes and companies in Germany, Q.Link.X is founded by the German Federal Ministry of Education and Research (BMBF) and aims at long-distance distribution of entanglement:
Quantum communication in a future quantum network [1] offers data encryption and transmission with physically verifiable security. The famous BB84 [2] protocol for sharing secret keys is limited to fiber lengths of 200-300 km [3]. Our goal is to distribute entanglement over a fiber based quantum network between different QR-nodes. With a chain of QR-nodes and using an entanglement swapping protocol [4] we aim to overcome the length limitation. The quantum channel is subdivided into several segments, each of them connected by a QR-node, as elementary, scalable and fiber based units.
In the subproject in Mainz, we collaborate within Q.Link.X on the platform of color centers in diamond for a QR. A fiber based optical cavity (Fig. 1) serving as an interface between single photons and a single color center in diamond, which serves as local quantum memory. Specifically, we employ a negatively charged Silicon-Vacancy Center (SiV-) in diamond, providing a narrow, stable and efficient emission in the zero-phonon line (ZPL) at 737 nm and long-lived electronic spin states [5,6]. A spin coherence time of >10 ms [7] is sufficiently long for QR applications, but is obtained only for temperatures well below one Kelvin. Thus, we will cool the diamond sample with a He3/He4 dilution refrigerator to temperatures below 100 mK.
Efficient coupling of the SiV- to the quantum channel is achieved with a fiber based microcavity, produced in collaboration with David Hunger's group from KIT. The cavity consists of a concave fiber facet [8], coated with a DBR stack and a plane mirror. The microcavity puts tight spatial boundaries to the vacuum light field, causing the SiV- center to emit a photon into the cavity mode (Purcell enhancement). SiV- embedded in thin diamond membranes, provided by Steven Prawer's group in Melbourne [9,10] are bound to the planar mirror. The position of the plane sample mirror can be controlled by nanopositioners, which allow for positioning of SiV- into the cavity mode (Fig. 2).
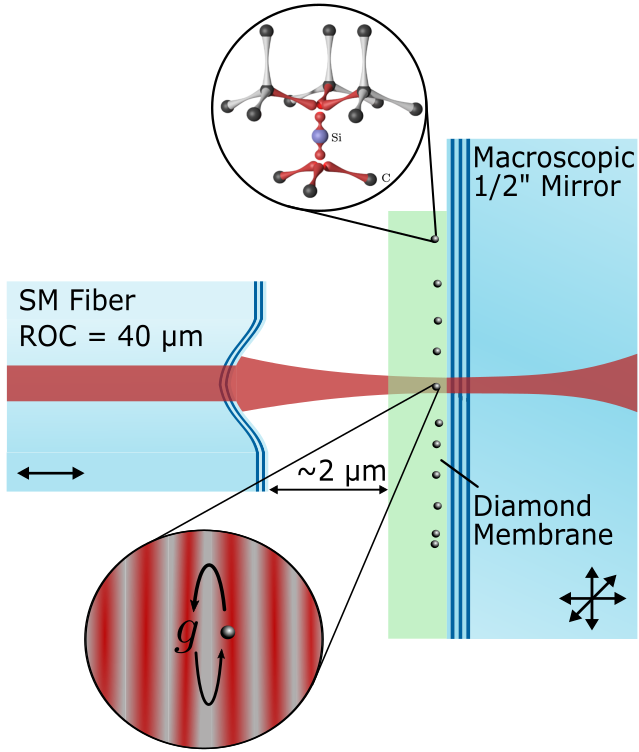
References:
[1] H. J. Kimble, Nature 453 (2008): Nature[2] C. H. Bennett et al., Proc. IEEE Int. Conf. on Com., Sys., and Sig. Process. (1984): ScienceDirect
[3] M. Takeoka et al., Nature Comm. 5, 5235 (2014): Nat. Comm.
[4] H.-J. Briegel et al., Phys. Rev. Lett. 26, 81 (1998): PRL
[5] T. Müller et al., Nat. Comm. 5, 3328 (2014): Nat. Comm.
[6] L. J. Rogers et al., Phy. Rev. Lett. 113, 262302 (2014): PRL
[7] D. D. Sukachev et al., Phys. Rev. Lett. 119, 223602 (2017): PRL
[8] J. Benedikter et al., Phys. Rev. App. 7, 024031 (2017): PRA
[9] A. Piracha et al., Nano Lett. 16, 5 (2016): Nano Lett.
[10] A. Piracha et al., Nanoscale 8, 12 (2016): Nanoscale
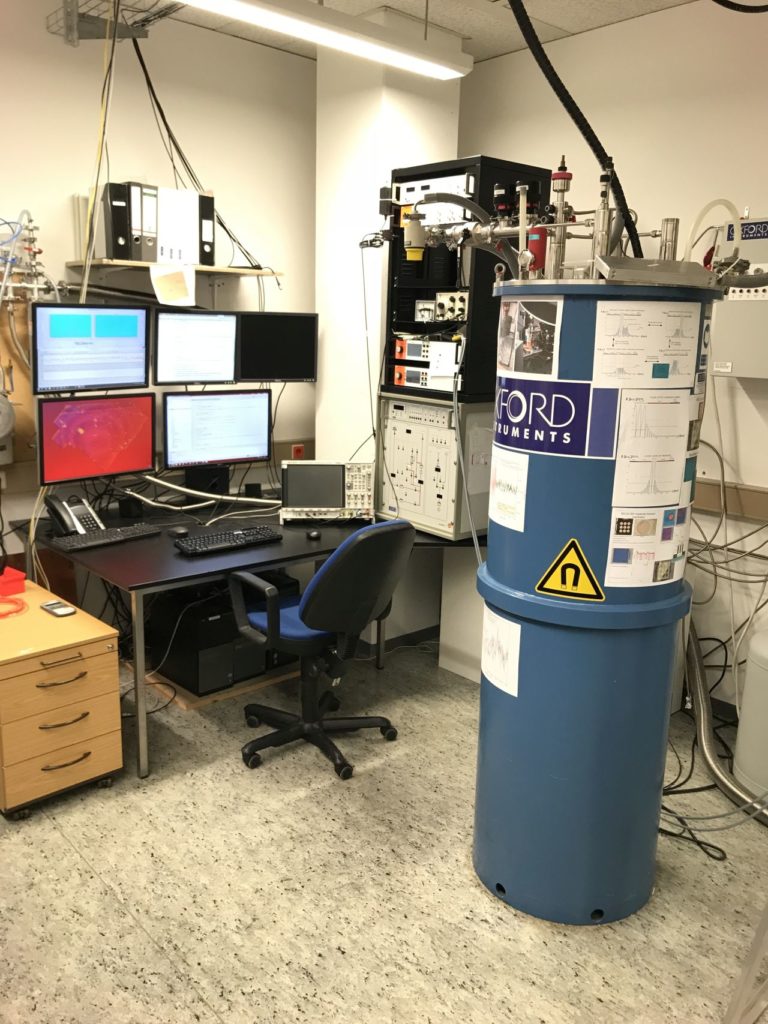